Daily Newsletter
August 28, 2012 - Protein Folding
Yesterday's newsletter focused on amino acids, how these monomers are polymerized, and the importance of their R (functional) group. Today we are going to further examine the importance of functional groups by discussing how they help form the working shape of a protein by causing a chain of amino acids to fold.
The primary structure of a protein is a chain of amino acids. Due to how proteins form, one end of the chain will end in an amino group (N-terminus), which the other end will have a carboxyl group (C-terminus). In Between these two terminal points will be a wide variety of amino acids.
The side chains of neighboring amino acids will begin to interact. They could be pulled toward each other, be repelled, or have nothing happen. Remember that the functional groups can twist around the chiral (central) carbon of the amino acid, so repulsion may just force the side chains to opposite sides of the chain (remember, you are dealing with 3-D structures here). The amino groups and carboxyl groups, even though they are part of the back bone, also retain polarity. Thus they can also be involved in the folding.
These interactions start the formation of the secondary level of protein structure. The two most common types of secondary structures are the alpha helix and the beta pleated sheet. These two types of secondary structures will help explain how the amino acid side chains start the folding process.
The α-helix relies on neighboring amino acids. Through the polarity of amino and carboxyl groups, the backbone of the molecule begins to twist and hold due to electrostatic interaction (van der Waals forces). The image to the left is an example of an alpha helix. The alpha represents the direction of the twist, and you will learn more of the naming of these in organic chemistry.
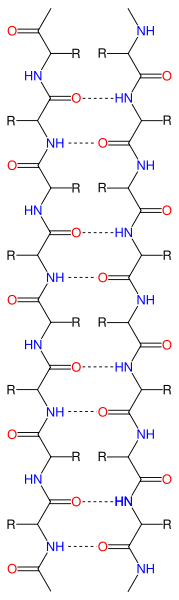
In contrast, β-pleated sheet, shown on the right, has interactions between different regions of the primary structure. While only one amino acid chain is involved, in this case the chain is not twisting. Instead, neighboring regions become attracted to each other. Also, the electrostatic interaction is between amino and carboxyl groups, not R groups. Multiple regions can be brought together to form these sheets as indicated in the diagram below.
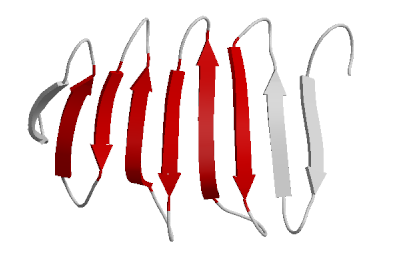
Notice that with the α-helix and β-pleated sheet we have altered the structure of the protein. It is no longer a linear chain, but has greater dimensionality. We have either turned the protein into a rope/cord (α-helix) or into a "plane" with two faces(β-pleated sheet). Now areas that were once distant have been brought closer together. Now side chains can start interacting with each other.
In the tertiary structure, different regions of the protein are brought into association. Electrostatic and hydrophobic interactions will force more conformational (structural) changes onto the protein. This will lead to a 3-dimensional structure. In the following diagram to the left, you can see an example of

a protein in primary and then tertiary structure.
Notice that the secondary structures are visible, but even these have been folded into each other.
The forces that govern this are found in the R (functional) groups of the amino acids. Hydrophobic areas cluster together. Positive and Negative charges attract, while like charges repel. Polar partially charged side chains further interact, either with each other, or with full charged. We also have a new interaction.

Many proteins are functional at the tertiary structure. Here you will see either globular or linear proteins

NOTE: Some proteins are functional in the tertiary structure, but others are only functional when you have multiple individual proteins forming the quatrenary structure.
Denaturation: The protein is held together primarily through electrostatic interactions. What happens when a protein warms up? It starts to unfold. Why? The electrostatic interactions weaken as kinetic movement of the atoms increases. Acids and bases, with their charged H+ and OH- also disrupt these electrostatic interactions. Secondary and tertiary structures begin to change conformation. Most notably, they unfold. To denature a protein is to unfold it....but....
What if you only apply a mild heat, let's say your muscles warm up due to exercise. What happens to the proteins? What happens to hemoglobin when it passes through a warm temperature? Your muscles are also metabolizing, and as we will see, produce acids. What does this do? So, is denaturation all or nothing?
The tertiary and quatrenary structures all have a specific electrochemical profile.
What happens if you add a charged particle/compound to a protein? What happens if I add a new positive charge? Answer: The protein will change shape (conformation).
What will this due to the function of the protein? It could actually active the protein, but it could also deactivate the protein. This will be a discussion a little later in the semester, but I want you to start thinking of the implications.
Administrative Note:
From now on, there will be a forum for each challenge.Make sure that you rate the posts of your fellow learners.
I try to hit as many posts as I can, but I will miss some throughout the semester. Remember, you can always come talk with me if you are uncertain about a post.
No comments:
Post a Comment