Daily Newsletter
September 30, 2013 - Glycolysis steps 1-5
Today we start looking at the metabolic pathway of glycolysis. Recall that in catabolism we are harvesting carbon (heterotroph) and energy (chemotroph). Moment by moment, cells are having to determine whether they have a need for carbon or energy. Intermediates from our catabolic pathways provide precursors for other biochemicals, but they also provide energy in the form of reducing potential. Reducing potential can be used for anabolic reactions, but will also be used to generate ATP. Remember: catabolic pathways will yield carbon precursors, reducing power (potential), and ATP.
With digital technology, you can go on line and pull down images of the glycolytic pathway. Nearly every biology textbook has these images. Your goal this weak is NOT to memorize these metabolic reactions. Your goal is to understand what is happening. If you have an idea of how to read a metabolic pathway, then you can look at any pathway given to you and have an understanding of what is happening. To do this, you need become familiar with enzyme names and common reactions. As you take organic chemistry, your ability to read metabolic pathways will improve, but remember you first need a foundation (this is your foundation).
Glycolysis
Glycolysis describes the splitting of glucose into two three-carbon Pyrvate molecules. This pathway consists of 10 reactions that carry out this splitting by inducing specific changes into the molecule. The first five steps are classified as preparatory, or energy consumptive. In these steps, we are preparing the molecule of glucose for the first split.Glucose is chemical stable. Glucose does not spontaneously explode or degrade. Chemical stability also implies that it does not react easily. So, we need to make it more reactive. We also need to get it into the correct configuration for splitting. That is the goal of the first four steps.
In step 1, we use the enzyme hexokinase (specifically Glucokinase). The root word here is kinase. A kinase is an enzyme that adds a phosphate group to a molecule. In this case, hexokinase is an enzyme that adds a phosphate group to a six carbon sugar, in this case glucose. You will notice in the above picture that the enzyme is referred to as glucokinase. Glucokinase is a specific hexokinase (Hexokinase IV), and is found in specific mammalian cells such as the intestines, liver, pancreas, and brain.(Recall, the hydrolysis of the terminal phosphate of ATP is coupled with the covalent bonding of the phosphate to the substrate).
Hexokinase is generally attached to the glucose carrier found in the cell membrane. When glucose is brought into the cell, hexokinase adds a phosphate group to the sixth carbon. Glucose 6-P refers to a glucose molecule with a phosphate on the sixth carbon. Glucose and Glucose 6-P are different molecules, with their own concentration gradients. By doing this, we remove glucose from the inside of the cell (it has become Glucose 6-P). As such, there is always a glucose concentration gradient (outside high, inside low).
In order to carry out this phosphorylation, we use ATP. ATP transfers the terminal phosphate to the sixth carbon of glucose via a coupled reaction. (enzymatically, how would this happen? Would you need both ATP and Glucose in the active site?)
Why do we need to phosphorylate glucose? This is an important question, and something you should ask for every metabolic reaction. Why do we need to do it? What is the end product? You should also ask yourself questions about the enzyme.
- Why do you phosphorylate glucose?
- Glucose is stable, so the addition of phosphate with its -2 charge causes an electrical instability in the molecule.
- Glucose 6P is more reactive than Glucose.
- Glucose 6P can not leave the cell through the Glucose Carrier (they are different molecules now).
- Glucose 6P does not interfere with the concentration gradient of Glucose (they are different molecules, each with a concentration gradient).
- Glucose then remains high on the outside of the cell, but almost zero inside of the cell (incredibly strong concentration gradient).
- What about the enzyme?
- Is it regulated?
- Unidentified allosteric regulation.
- What is the structure?
- 465 amino acids
- What does the active site look like?
- Active site fits Glucose and ATP.
- What about the activity?
In the second reaction of glycolysis, the enzyme phosphoglucose isomerase (Glucose-6-phosphate isomerase) causes the glucose molecule to rearrange slightly into the the hexose isomer fructose.
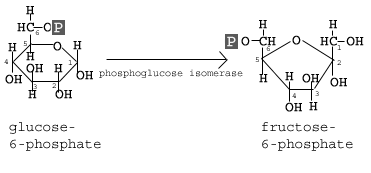
The ΔGo' for this reaction is +0.4 kcal/mol; so in a lab it is not expected to proceed at significant levels. Enzymes though allow for this reaction to proceed at significant rates. Of course, a build up of fructose-6-phosphate would disrupt the dynamic equilibrium we want, so we move this product to become the substrate of the next reaction.
Reaction 3 is catalyzed by phosphofructokinase. This enzyme will add a phosphate group onto the 1st carbon, and the reaction has a ΔGo' of -3.4 kcal/mol.
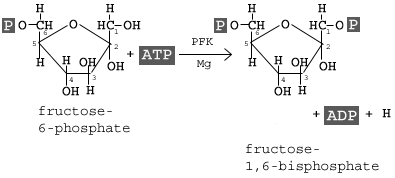
Now look at the resulting molecule: Fructose 1,6-bis phosphate. There are two carbons sticking out of the ring structure. In the image, you are seeing them on the same plane (above the ring). The phosphates have a -2 charge. What are they doing to the molecule?

In the fourth reaction, we see the splitting of the glucose molecule. The molecular tension is focused on the covalent bond between the 3rd and 4th carbon. Aldolase is the enzyme that catalyzes this reaction. This reaction deals with some specific organic chemistry, so we will hold off on the full discussion at this time.
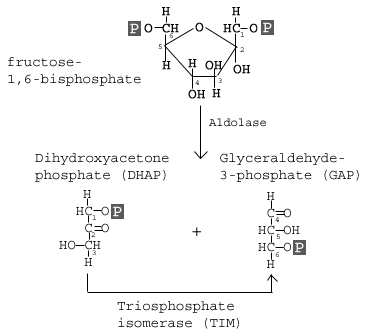
NOTE: the ΔGo' of reaction 4 is generally reported at +5.7 kcal/mol. Remember that these numbers are from isolated reactions. Covalent bonds are strong and stable; even with the molecular tension of 2 phosphate groups, the bond between carbon 3 and 4 does not want to break. That is where the enzyme aldolase comes in. If you remember from lecture, we talked about what can happen at an active site. One of the options was inducing physical stress and charges. What would happen if you had complementary positive charges for the phosphate pulling away from the fructose? What would happen if you physically pulled these regions apart? Would it be easier to break the covalent bond?
In reaction 5, we convert DHAP into a more usable form, namely Glyceraldehyde 3-Phosphate (G3P is a common acronym). The enzyme that carries out this reaction is shown in the above diagraph: Triosphosphate Isomerase.
At the end of these first five steps, two phosphates have been added to a hexose in order to destabilize the sugar and cause it to break in a very specific manner (between carbon 3 and 4). The result is that we have two 3-carbon sugars: G3P. Notice the oxidation state of the molecule. There are a number of hydrogens attached directly to carbon. There is energy that we can harvest in the form of reducing potential. Tomorrow we will continue with steps 6-10.
Daily Challenge
In your own words, describe steps 1-5 of glycolysis. Discuss how the enzymes make the reactions possible, including how they can couple reactions (this is important for any reaction with ATP). Discuss how the stepwise change in the molecule allows for the localization of the bond that needs to be broken. Why don't we just break that bond immediately? Remember, this will become part of your next milestone paper.Due October 2, 2013 at 11:55pm.
No comments:
Post a Comment